Abstract
Background
Abstract
The severe acute respiratory syndrome (SARS) has recently been identified as a new clinical entity. SARS is thought to be caused by an unknown infectious agent
Methods
Clinical specimens from patients with SARS were searched for unknown viruses with the use of cell cultures and molecular techniques.
Results
A novel coronavirus was identified in patients with SARS. The virus was isolated in cell culture, and a sequence 300 nucleotides in length was obtained by a polymerase-chain-reaction (PCR)–based random-amplification procedure. Genetic characterization indicated that the virus is only distantly related to known coronaviruses (identical in 50 to 60 percent of the nucleotide sequence). On the basis of the obtained sequence, conventional and real-time PCR assays for specific and sensitive detection of the novel virus were established. Virus was detected in a variety of clinical specimens from patients with SARS but not in controls. High concentrations of viral RNA of up to 100 million molecules per milliliter were found in sputum. Viral RNA was also detected at extremely low concentrations in plasma during the acute phase and in feces during the late convalescent phase. Infected patients showed seroconversion on the Vero cells in which the virus was isolated.
Conclusions
The novel coronavirus might have a role in causing SARS.
The severe acute respiratory syndrome (SARS) was recently identified as a new clinical entity.1,2 Patients present with fever, dry cough, dyspnea, headache, and hypoxemia. Typical laboratory findings are lymphopenia and mildly elevated aminotransferase levels. Death may result from progressive respiratory failure due to alveolar damage.3 SARS appears to be caused by an unknown infectious agent that is transmitted from human to human. The World Health Organization (WHO) had recorded 2353 cases by April 4, 2003. About 4 percent of patients with SARS have died.4 The SARS epidemic started in Asia, with the majority of cases occurring in China and the Asia–Pacific region. The epidemic has spread from Asia to other continents through international travel. The WHO has established a network of international laboratories to facilitate the identification of the causative agent of SARS. As part of this network, we have identified and characterized a novel coronavirus in patients with SARS.
Methods
Patients and Samples
The index patient was a 32-year-old male physician. From March 3 to 9, 2003, he treated a patient with atypical pneumonia in Singapore who had arrived from Hong Kong. On March 9 (day 1) his illness started with abrupt onset of fever (temperature, 39.4°C), when he was in New York. On day 4, a dry cough and a sore throat developed, along with erythema on the trunk. During a stopover in Frankfurt, Germany, on his flight to Singapore, he was transferred to an isolation unit at the Frankfurt University Hospital with suspected SARS (day 7). He had hypoxemia (partial pressure of oxygen in arterial blood, 59 mm Hg) and required oxygen supplementation. Chest radiography showed worsening bilateral opacifications. Laboratory abnormalities included elevated levels of aspartate aminotransferase (46 U per liter) and lactate deydrogenase (478 U per liter) and an elevated maximal C-reactive protein level (30.7 mg per deciliter), as well as leukopenia and lymphopenia. Convalescence began on day 10. Treatment involved levofloxacin, vancomycin, imipenem, doxycycline, and oseltamivir. Two contacts of the index patient (Contacts 1 and 2) were isolated as well. Contact 1, the patient's wife, reported a headache after admission (March 15, day 1). Fever (temperature, 38.2°C) and myalgia developed on day 2. The temperature decreased during days 5 and 6, but on day 7 fever developed again and there were crackles over her lungs, accompanied by dry cough and hypoxemia (partial pressure of oxygen in arterial blood, 65 mm Hg). Convalescence began on day 9. Laboratory abnormalities included a maximal C-reactive protein level of 1.5 mg per deciliter, leukopenia, and lymphopenia. Therapy comprised erythromycin and ceftriaxone. Contact 2, the mother of Contact 1, had headache and myalgia on March 13 (day 1) and sore throat and fever (temperature, 38.2°C) on day 2. Fever disappeared on day 3. She reported burning pain in the eyes on days 4 and 5. There were no pathologic findings on chest radiography. Pathologic laboratory findings included a maximal C-reactive protein level of 9.9 mg per deciliter, an increase in the aspartate aminotransferase level to 154 U per liter, and an increase in the lactate dehydrogenase level to 319 U per liter between days 9 and 16. Therapy involved imipenem, levofloxacin, doxycycline, and oseltamivir. A total of 49 specimens from 18 patients with suspected or probable SARS, according to the WHO case definition, and from 21 healthy contact persons were sampled between March 5 and March 27, 2003, during the SARS epidemic in Hanoi, Vietnam.2 A total of 54 stool samples from patients in Germany were available as controls. Microbiologic and Virologic Testing for Typical and Atypical Respiratory Pathogens Respiratory and blood specimens from the patients in Frankfurt were tested by polymerase chain reaction (PCR) with specific primers for Mycoplasma pneumoniae, Chlamydia pneumoniae, human cytomegalovirus, adenoviruses, respiratory syncytial virus, parainfluenzavirus types 1, 2, 3, and 4, Hendra virus, Nipah virus, human metapneumovirus, influenzaviruses A and B, rhinovirus, and human coronavirus strains OC43 and 229E, as well as with universal primers for herpesviruses, arenaviruses, bunyaviruses, enteroviruses, alphaviruses, flaviviruses, filoviruses, and paramyxoviruses. Respiratory specimens were also tested by antigen enzyme-linked immunosorbent assay (ELISA) for M. pneumoniae, influenzaviruses A and B, and respiratory syncytial virus. Antigen ELISA for legionella species was performed with urine. Paired serum samples were tested serologically for C. pneumoniae (IgA and IgG), C. trachomatis (IgA and IgG), C. psittaci (IgG), M. pneumoniae, Coxiella burnetii, influenzaviruses A and B (IgG and IgA), dengue virus (IgG and IgM), measles virus (IgG and IgM), hantaviruses, adenoviruses, parainfluenzavirus types 1, 2, and 3, and respiratory syncytial virus. Electron microscopy was performed with negative staining of respiratory and blood samples. In addition, cells in bronchoalveolar-lavage fluid were analyzed with the use of ultrathin sections embedded in Epon and LR Gold resins. Vero, Madin–Derby canine-kidney, and A549 cells were inoculated with respiratory and blood specimens from all three patients and grown under biosafety level 3 or 4 conditions. RNA Extraction Sputum samples were shaken for 30 minutes with an equal volume of acetylcysteine (10 g per liter) and 0.9 percent sodium chloride. The resulting homogenate, native body fluids, resuspended swab samples (in 1 ml of phosphate-buffered saline), or tissue-culture supernatant was extracted with a viral RNA kit (QIAamp, Qiagen; elution volume, 60 μl). Stool specimens were extracted with the QIAamp stool kit (Qiagen). Random Reverse-Transcriptase–PCR Procedure A volume of 2 μl of RNA solution was analyzed with a random reverse-transcriptase (RT)–PCR assay. The Superscript II platinum Taq polymerase one-step RT-PCR kit (Invitrogen) was used for the reaction (20 μl total volume). Reactions contained 10 μl of buffer concentrate, 2 mM of magnesium sulphate, 0.8 μl of enzyme mixture, and 1.9 μM of each of two primers. Fifteen primer pairs were used. Some of the primers contained degenerate positions, and most had a thymidine residue at their 3' ends to allow DNA polymerase to work in spite of incomplete nucleotide matches at the ends of the primers.4 The primers were originally designed to target the genome of yellow fever virus strain 17D and the polymerase gene of Paramyxoviridae. Thermal cycling comprised 42°C for 30 minutes; 95°C for 3 minutes; 10 cycles of 95°C for 10 seconds, 55°C for 15 seconds (decreasing by 1°C per cycle), 72°C for 40 seconds; 40 cycles of 95°C for 10 seconds, 56°C for 10 seconds, and 72°C for 40 seconds. Products were analyzed on a 1 percent agarose gel, gel-purified, and reamplified with the use of the corresponding primers but without degenerate positions. Products were sequenced with the use of a didesoxy terminator sequencing reaction (BigDye terminator reaction mix, Applied Biosystems) and an automated DNA sequencer (model 3100, Applied Biosystems). RT-PCR Specific for the Novel Coronavirus Table 1. Primers and Protocols for Diagnostic Polymerase-Chain-Reaction Assays.For diagnostic RT-PCRs, the Superscript II platinum Taq polymerase one-step RT-PCR kit was used. Details of the procedures are summarized in Table 1. An RNA standard transcribed in vitro was generated by amplification of the target region with primers BNIoutS2 and BNIoutAs. The fragment was cloned and transcribed into RNA in vitro, essentially as described elsewhere.5 Sequence and Phylogenetic Analysis We searched for homologies to known sequences using the nucleotide or translated data base of the Basic Local Alignment Search Tool (BLAST) at http://www.ncbi.nlm.nih.gov:80/BLAST/. opens in new tab. Phylogenetic analysis was performed with the use of the Phylogeny Inference Package (PHYLIP), version 3.57c.6
Results
Microbiologic Testing for Common Pathogens A large number of tests for known respiratory pathogens were performed with specimens from all three patients in Frankfurt. The test results were negative, except as follows. Paramyxovirus-like particles were seen in throat swabs and sputum samples from the index patient by electron microscopy. The particles were scarce. However, several PCR tests specific for virus species of the family Paramyxoviridae were negative (including tests for human metapneumovirus), as were PCR assays based on primers designed to react broadly with all members of that family. C. pneumoniae was not detected by PCR or antigen ELISA in sputum of the index patient from day 9. However, on day 11, electron microscopy of cells in a bronchoalveolar-lavage specimen from the index patient showed a severe intracellular bacterial infection, and the bronchoalveolar-lavage cells reacted in immunofluorescence analyses with a monoclonal antibody directed against C. pneumoniae. Consistent with this finding, there was an increase by a factor of four in the C. pneumoniae IgA titer in the index patient between day 10 and day 13. Isolation and Characterization of a Novel Coronavirus After six days of incubation (on March 21), a cytopathic effect was seen on Vero-cell cultures inoculated with sputum obtained from the index patient on day 7. Twenty-four hours after a single passage, nucleic acids were purified from the supernatant. Random amplification was performed with 15 different PCRs under low-stringency conditions. We had previously shown that this method is able to detect unknown pathogens growing in cell culture (unpublished data). To detect RNA viruses, an initial reverse-transcription step was included.
About 20 distinct DNA fragments were obtained and sequenced. The resulting sequences were subjected to BLAST data-base searches. Most of the fragments matched human chromosome sequences, indicating that genetic material of the cultured cells had been amplified (Vero cells are derived from monkeys). Three of the fragments did not match any nucleotide sequence in the data base. However, when a translated BLAST search was performed (comparison of the amino acid translation in all six possible reading frames with the data base), these fragments showed homology to coronavirus amino acid sequences, indicating that a coronavirus had been isolated. Two of the fragments were 300 nucleotides in length and identical in sequence, and the third fragment was 90 nucleotides in length (sequences BNI-1 and BNI-2, respectively, as reported on the Web site of the WHO network on March 25) (Figure 1A). Detailed sequence analysis revealed that both fragments were located in the open reading frame 1b of coronaviruses and did not overlap with a 400-nucleotide coronavirus fragment identified by colleagues at the Centers for Disease Control and Prevention (CDC) (sequence CDC, reported on the Web site of the WHO network on March 24) (Figure 1A). There were sequences of six coronaviruses of all phylogenetic lineages (groups 1, 2, and 3) in the sequence data base that overlapped with sequences BNI-1 and BNI-2. All known sequences were only distantly related to the new sequences (Figure 1B). The BNI-1 nucleotide sequence diverged from the known sequences by between 39 percent (bovine coronavirus) and 46 percent (porcine epidemic diarrhea virus). Such distances are typically found between different genetic lineages of coronaviruses.7 A stable phylogeny was obtained with the BNI-1 fragment, indicating that the isolated coronavirus segregates between genetic groups 2 and 3 (Figure 1C). The novel coronavirus isolate was termed FFM-ic (for Frankfurt am Main index case). To compare our isolate with that obtained by the CDC, a fragment corresponding to the CDC fragment was amplified from the cell-culture supernatant and sequenced. Both sequences were 100 percent identical. Next, primers specifically targeting the BNI-1 fragment and the CDC fragment were synthesized. With the use of a long-range PCR protocol,8 a region extending from the CDC fragment to the BNI-1 fragment was amplified. The obtained fragment had the expected length of 3 kb. It was sequenced from both ends, and the sequences were found to be identical to the CDC and BNI-1 sequences, respectively, demonstrating that the two sequences were derived from a contiguous RNA molecule and, thus, from the same virus. Since the same virus was isolated from two independent cases of SARS, and since there was serologic evidence of an acute infection with this virus in our index patient and also in Contact 1 (as described below), it was considered that the coronavirus might have a role in causing SARS. Specific diagnostic assays were therefore established for the detection of the pathogen. Establishment of Diagnostic PCR Assays Targeting the BNI-1 Fragment Table 2. Detection of the Novel Coronavirus in Various Clinical Specimens with the Use of Different PCR Assays.Figure 2. Real-Time Polymerase Chain Reaction (PCR) Targeting the BNI-1 Fragment of the Novel Coronavirus.A nested set of primers was designed within the BNI-1 fragment (Figure 1B). The outer set of primers (protocol 3) (Table 1) detected the virus in clinical specimens from the index patient and Contact 1, who also had clinical signs of SARS (Table 2). Additional specimens were positive on nested RT-PCR (protocols 3 plus 5) (Table 1 and Table 2). (The availability of PCR protocols at http://www.bni.uni-hamburg.de/. opens in new tab was reported to the WHO network on March 27 and to ProMed mail [an international e-mail notification service for infectious-disease outbreaks] on March 30.) To have a practical and quantitative test, a real-time RT-PCR with a 5'-nuclease probe was established (protocol 6) (Table 1 and Figure 1B). After optimization with the use of quantified RNA transcribed in vitro, the assay reliably detected 10 copies of RNA per reaction, corresponding to 830 RNA molecules per milliliter of specimen (Figure 2). The sensitivities of nested and real-time PCR were equivalent (Table 2). The specificity of the PCR with outer primers, of the nested PCR, and of the real-time PCR (protocols 3, 3 plus 5, and 6, respectively) (Table 1) was tested with the use of RNA purified from cultures of bovine coronavirus, avian infectious coronavirus, porcine transmissible gastroenteritis coronavirus, and human coronaviruses 229E and OC43. None of the PCR assays cross-reacted with these viruses — a finding that is consistent with their considerable genetic differences from the novel coronavirus. Quantification of the viral RNA concentration in clinical specimens from the index patient and Contact 1 by real-time PCR revealed that the highest concentration — as high as 100 million copies per milliliter — was present in sputum (Table 2). After enrichment of virus by ultracentrifugation, viral RNA was also detected in the serum of the index patient, indicating the presence of low-level viremia during symptomatic disease. Both the index patient and Contact 1 had viral RNA in stool samples obtained during late convalescence, suggesting that virus may be shed in feces for prolonged periods. To investigate whether the novel coronavirus was prevalent in patients in Germany who had gastrointestinal symptoms, a collection of 54 stored stool samples was tested with the use of the real-time RT-PCR assay. None of the samples tested positive. The established PCR assays have also been used to test respiratory samples from German patients with symptoms and a travel history compatible with SARS. So far, 67 samples from 55 patients have been tested. One patient fulfilling the WHO criteria for probable SARS was coronavirus-positive on PCR. PCR protocols, as well as positive control material, have been made available to laboratories worldwide. Testing of a Panel of Specimens from Patients from Asia with SARS Table 3. Proportion of Patients with a Positive RT-PCR Result for Coronavirus.To provide evidence for the hypothesis that the novel coronavirus is associated with SARS, further specimens from patients with probable and suspected SARS, as well as healthy contacts of patients affected by the SARS epidemic in Hanoi, Vietnam, were tested by nested PCR assays targeting the CDC and BNI-1 fragments (protocols 2 plus 4 and 3 plus 5, respectively) (Table 1). The prevalence of the virus was 100 percent among patients with probable cases of SARS and 23 percent among those with suspected cases of SARS, whereas virus was not detected at all in the healthy contacts (Table 3). These preliminary data may point to an association between the novel coronavirus and SARS. A total of 15 of the PCR products were sequenced — 7 from the CDC fragment and 8 from the BNI-1 fragment. All sequences were 100 percent identical to the corresponding CDC and BNI-1 sequences, providing evidence of an epidemiologic link among these patients. Furthermore, these findings suggest that the virus is rather stable genetically. Serologic Response The serologic response to the coronavirus was tested by a standard immunofluorescence technique with serial serum samples from all three patients and coronavirus FFM-ic–infected Vero cells. Specific IgG was not detected in serum from the index patient and Contact 1 until day 9 and day 10, respectively. Thereafter, the IgG titer increased to 1:1500 in the index patient and 1:250 in Contact 1. No antibody response developed in Contact 2.
Discussion
The principal finding of the study is the identification of a novel coronavirus in patients with SARS. It appears that patients with SARS are acutely infected with this virus, since they have virus-specific IgG seroconversion. The high rate of positivity among patients with probable cases during an outbreak of SARS in Hanoi, in conjunction with the complete negativity among all healthy contacts of patients affected by the same outbreak, provides evidence of an association between the disease and the presence of this novel virus. The involvement of a coronavirus in a respiratory disease would not be without precedent: the two human coronaviruses are known to cause mild respiratory illness.9 One should bear in mind, however, that in the past, viruses have been initially isolated from patients with a specific disease but subsequent investigations revealed no actual association at all.10,11 Thus, larger studies with appropriate control groups are needed to verify or eliminate our hypothesis about the cause of SARS. The assays that have been established provide an excellent tool for such studies. It should also be taken into account that antigen that was present in primary cultures was used to detect the antibody response, and it remains to be firmly established that this response is indeed directed against the novel coronavirus rather than against an unknown agent that might have been isolated simultaneously. This possibility could be tested with the use of plaque-purified virus or recombinant proteins as antigen. By testing for a broad range of known pathogens, we also obtained evidence for infection with paramyxoviruses and C. pneumoniae. Paramyxoviruses — in particular, human metapneumovirus, which was previously implicated in SARS12 — could be largely ruled out by further investigation. Infection with chlamydia was confirmed in several assays. However, chlamydia was not found in other patients with SARS.3 Hence, it remains unclear whether these pathogens have a role as causative factors or cofactors in SARS. The quantitative analysis of various clinical specimens from the patients with SARS reveals interesting features of the viral infection. First, the viral RNA concentration in sputum was high in both patients, suggesting that shedding of the virus from the respiratory tract could be the primary route of transmission. The extremely high RNA concentrations found in sputum from the index patient would be consistent with a high level of contagiousness of the SARS agent. The detection of low amounts of viral RNA in serum from the index patient on day 9 would be compatible with a long viremic phase, suggesting that replication does not occur only in the respiratory tract. The elevated levels of aspartate aminotransferase and lactate dehydrogenase3 indeed suggest that the agent causing SARS is also replicating outside the respiratory tract. The presence of viral RNA in stool of the patients late during convalescence is reminiscent of characteristics of other coronaviruses.13 Shedding of the virus in feces may be an additional source of spreading, provided that the virus is stable in this environment. From a diagnostic point of view, it is important to note that nasal and throat swabs seem less suitable for diagnosis, since these materials contain considerably less viral RNA than sputum, and the virus may escape detection if only these materials are tested.
Supported by a grant (325-4539-85/3) from the Bundesministerium für Gesundheit and a grant (E/B41G/1G309/1A403) from the Bundesamt für Wehrtechnik und Beschaffung. Studies conducted at the Institute of Medical Virology in Frankfurt were supported in part by the Ministry of Science and Arts of the federal state of Hessen, Germany. Drs. Drosten and Günther contributed equally to this article. This article was published at www.nejm.org on April 10, 2003. We are indebted to the medical staff of the hospital in Hanoi, Vietnam, for providing specimens from and clinical data for their patients; to Britta Liedigk, Gaby Bauer, Marhild Kortenbusch, Valérie Lorin, Claudine Rousseaux, Maryse Tardy-Panit, Saliha Azebi, Christophe Batejat, Gilberte Coralie, and Aurélien Brionne for excellent technical assistance; to Uli Lass, Christina Schiel, and Olfert Landt (Tib-Molbiol, Berlin) for rapid service in oligonucleotide synthesis; to Volker Thiel and John Ziebuhr (University of Würzburg) for providing human coronavirus 229E; to Georg Herrler (Tierärztliche Hochschule, Hannover) for providing bovine coronavirus, avian infectious coronavirus, porcine transmissible gastroenteritis coronavirus, and human coronavirus OC43; and to Michèle Bouloy and Annette Martin for providing culture cells.
Author Affiliations
From the Bernhard Nocht Institute for Tropical Medicine, National Reference Center for Tropical Infectious Diseases, Hamburg (C.D., S.G., M.P., K.G., S.K., S.M., S.V., H.S.); the Institute of Medical Virology (W.P., H.R., A.B., J.C., M.S., H.W.D.) and the Medical Clinic III (H.-R.B., V.R.), Johann Wolfgang Goethe University, Frankfurt am Main; and the Institute of Virology, Philipps University, Marburg (S.B., L.K., M.E., H.-D.K.) — all in Germany; the Pasteur Institute, Molecular Genetics of Respiratory Tract Viruses, National Influenza Center (Northern France), Paris (S.W., A.-M.B., N.E., J.-C.M.); and the Institute of Virology, Erasmus University, Rotterdam, the Netherlands (R.A.M.F., A.D.M.E.O.).
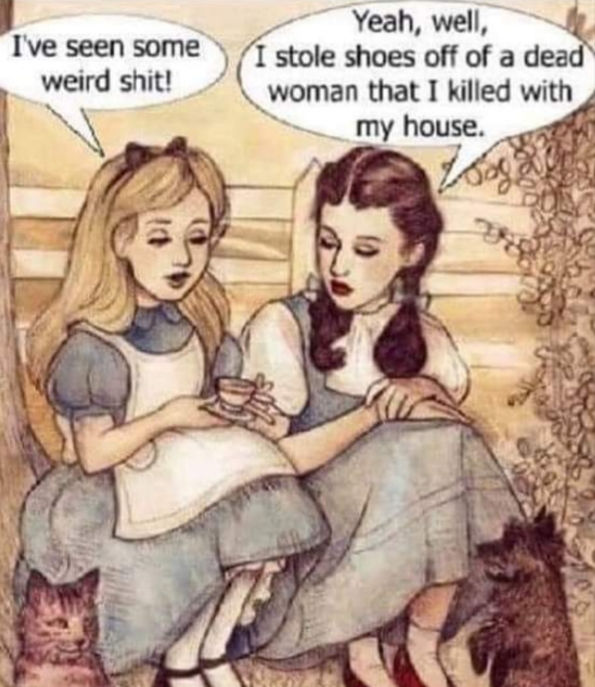